Pure science is not always easy to understand
READER COMMENTS
58(1)
Earth and Planetary Science Letters
Introduction
The Capitanian mass extinction, characterized by genus-level extinction rates of 42-61% (Stanley and Yang, 1994; Clapham et al., 2009; Stanley, 2016), was particularly severe for shallow-marine taxa including fusulinacean foraminifers, corals, bivalves, and ammonoids (Shen and Shi, 2009; Wignall et al., 2009; Bond et al., 2010). This event also affected terrestrial ecosystems and resulted in a mass extinction of land vertebrates (Retallack et al., 2006). The timing and number of episodes of the Capitanian biocrisis remain controversial, with proposals for either a mid-Capitanian (Wignall et al., 2009; Bond et al., 2010) or an end-Capitanian event (Shen and Shi, 2009; Shen et al., 2020). Although a plethora of mechanisms have been proposed for this extinction, including marine anoxia, volcanism, sea-level fall, ocean acidification, and climatic change (Zhou et al., 2002; Isozaki et al., 2007; Wignall et al., 2009; Bond et al., 2010, 2015, 2020; Zhang et al., 2015; Wei et al., 2016), no consensus regarding its causation has been reached to date.
Uranium isotopes in marine carbonates (Ucarb) permit quantitative reconstruction of secular variation in average global-ocean redox conditions. The basis for this proxy is the long residence time of U in seawater (∼400 kyr) relative to the oceanic mixing time (∼1-2 kyr for the modern) (Ku et al., 1977). Microbially mediated reduction of U(VI) to U(IV) under anoxic conditions at the seafloor results in a substantial decrease in U solubility in seawater (Weyer et al., 2008; Andersen et al., 2014). Because 238U is preferentially reduced and immobilized relative to 235U, the U of seawater decreases as its burial flux increases. Thus, a global increase in the extent of ocean anoxic seafloor is expected to cause simultaneous decreases in seawater U concentration [U] and U. Primary marine carbonates record the U of contemporaneous seawater with little fractionation, although precipitation of secondary carbonate cement in the sulfate reduction zone can lead to isotopic shifts of +0.2‰ to +0.4‰ in bulk-rock U (Chen et al., 2018; Tissot et al., 2018). Carbonate U records have been used to quantitatively reconstruct the extent of seafloor anoxia during several oceanic anoxic events (Lau et al., 2016; Song et al., 2017; Bartlett et al., 2018; Zhang et al., 2018).
Although several studies have used elemental or mineralogical proxies to examine local environmental redox variation in Middle Permian sections, with possible implications for ocean anoxic events during the mid-Capitanian (Bond et al., 2015, 2020) and end-Capitanian (Zhang et al., 2015; Wei et al., 2016), global-ocean redox proxies such as carbonate U have not yet been generated for this event interval. Here, we use the carbonate U proxy to document two discrete episodes of global-ocean anoxia during the Capitanian and demonstrate their synchronicity with the two phases of the Middle Permian mass extinction identified in earlier studies.
(2)
Palaeogeography, Palaeoclimatology, Palaeoecology
High-temperature combustion event spanning the Guadalupian−Lopingian boundary terminated by soil erosion
Introduction
As the most significant environmental crisis of the Phanerozoic, the Permian−Triassic mass extinction resulted in the largest crash in global biodiversity in Earth history, dramatically redirecting the course of evolution (Sepkoski, 1981; Erwin, 2006; Alroy et al., 2008; Chen and Benton, 2012; Fan et al., 2020). These severe losses reflect not only the mortality of most taxa at the end of the Permian (252 Ma), but also a decline process that started in the late Guadalupian (mid Capitanian −262 Ma) ∼10 million years earlier (Wignall et al., 2009a, Wignall et al., 2009; Bond et al., 2010, Bond et al., 2015; Zhang et al., 2019; Shen et al., 2020b). This was followed by the Guadalupian–Lopingian (G−L) boundary (GLB) event at 259 Ma (Wang et al., 2004; Kaiho et al., 2005; Chen et al., 2009; Shen et al., 2020b; Huang et al., 2021), reaching the minimum of marine animal species diversity at the GLB (Fan et al., 2020). While the late Guadalupian (late Capitanian) crisis is marked by significant declines in metazoan reef abundance and fusulinid diversity (Fluegel and Kiessling, 2002; Huang et al., 2019; Arefifard and Payne, 2020; Feng et al., 2020), marine extinctions during the latest Guadalupian to earliest Lopingian were not significant (Fan et al., 2020) as compared to the major terrestrial tetrapod extinctions at 260 Ma (late Capitanian) and 259 Ma (GLB) (Day et al., 2015; Marchetti et al., 2019), as supported by stage level statistical data (Sahney et al., 2010; Benton et al., 2013). The G−L transition event is one of main environmental-biotic events during the Capitanian (as well as the mid-Capitanian event) and is marked by a large carbon isotope excursion (Huang et al., 2019; this study), minimum marine animal diversity (Fan et al., 2020), and the maximum value of Hg/TOC (Grasby et al., 2016) spanning the GLB.
Although previously reported fossil data (Bambach, 2006; Stanley, 2016) showed high % values of marine biotic losses (48 and 34% in genus level, respectively) in the end of the Guadalupian, these are uncertain due to “smear back” (Signor-Lipps Effect) from the great end-Permian crisis, and are enhanced by the loss of sedimentary records related to the global low sea-levels during the G–L transition (Bambach, 2006). In contrast, the fossil data from the Laibin area, Guangxi Province, South China is unaffected by both the Signor-Lipps Effect and the sea-level low because they were derived from continuous GLB successions without a time gap (Kaiho et al., 2005; Chen et al., 2009; Huang et al., 2021). The South Chinese data showed a low-magnitude loss of marine invertebrates during the Capitanian including across the GLB (Fan et al., 2020), with 11% species loss except for brachiopods (Kaiho, 2022), although they recorded losses in biodiversity at generic and species levels similar to previous studies (on the end-Ordovician, Frasnian–Famennian boundary, and end-Permian mass extinctions [Sepkoski, 1996; Bambach, 2006; Stanley, 2016]). The species diversity of foraminifera and anthozoa minimized at 259 Ma (Fan et al., 2020). Global low sea-levels during the G–L transition resulted in mostly global unconformities in shallow depositional settings spanning the latest Guadalupian to earliest Lopingian interval, and as such the apparent magnitude of marine invertebrate extinction at the generic level appears higher than the true loss (Hallam and Wignall, 1999; Bambach, 2006; Chen et al., 2009; Huang et al., 2019; Li et al., 2023).
Previous studies interpreted that the late Guadalupian crisis, from the mid-Capitanian to end-Capitanian, could have been caused by a range of factors, including global regression (Hallam and Wignall, 1999; Isozaki, 2009a, Isozaki, 2009b; Chen et al., 2009), oceanic anoxia (Bond et al., 2015; Zhang et al., 2021) with oxic conditions in central Panthalassa (Fujisaki et al., 2019; Onoue et al., 2021), as well as environmental changes due to eruption of the Emeishan Large Igneous Province (ELIP) in South China (Wignall et al., 2009a; Isozaki, 2009a, Isozaki, 2009b; Bond et al., 2010, Bond et al., 2015; Zhang et al., 2019; Huang et al., 2019; Chen and Xu, 2019). In the Penglaitan section of the Laibin area, the global stratotype section (GSSP) for the GLB, mercury (Hg) concentration/total organic carbon (Hg/total organic carbon [TOC]) ratios show two spikes, interpreted as pulsed eruption of the ELIP over the G−L transition (Huang et al., 2019). Mercury concentration peaks were also detected at Kapp Starostin, Spitsbergen, during the late Guadalupian, starting from the mid-Capitanian and peaking during the G−L transition (Grasby et al., 2016).
While Hg records have been used to suggest volatile volcanism is concurrent with the G − L crisis, the source of mercury and the magnitude of environmental impact remains disputed. This is because Hg can be derived from soil erosion–oxidation (weathering) as well as volcanism (Grasby et al., 2013, Grasby et al., 2017, Grasby et al., 2019, Grasby et al., 2020; Wang et al., 2018, Wang et al., 2019; Derkowski and Marynowski, 2018; Shen et al., 2019, Shen et al., 2020a; Dal Corso et al., 2020; Racki, 2020; Kaiho et al., 2021a, Kaiho et al., 2021b, Kaiho et al., 2022). Localized mercury anomalies can also be generated by changes in local redox conditions or binding to clays. Thus, careful analyses are required before ascribing a Hg/TOC anomaly to a volcanic source (Sanei et al., 2012; Grasby et al., 2019; Them et al., 2019; Shen et al., 2019, Shen et al., 2020a).
Five- to seven-ringed polycyclic aromatic hydrocarbons (PAHs) are formed by organic matter combustion in typical wildfires, normal oil fires, and mudstones that have undergone a high degree of diagenesis (Wang et al., 1999; Marshall et al., 2007; Vergnoux et al., 2011; Karp et al., 2018; Song et al., 2020). The sum of five- to seven-ringed PAHs (benzo[e]pyrene, beozo[ghi]perylene, and coronene) are commonly dominate at mass extinction horizons (Arinobu et al., 1999; Nabbefeld et al., 2010; Kaiho et al., 2016a, Kaiho et al., 2016b, Kaiho et al., 2021a, Kaiho et al., 2021b, Kaiho et al., 2022; Lyons et al., 2020; Fox et al., 2022), although the proportion of coronene is largely different in each mass extinction (from 0.1 to 0.9) (Kaiho et al., 2021a, Kaiho et al., 2021b, Kaiho et al., 2022). High seven-ringed coronene contents are another important geochemical proxy indicating volatile volcanism or projectile impacts, as it is formed by high temperature combustion (>1200 °C; Norinaga et al., 2009; Kaiho et al., 2021a, Kaiho et al., 2021b, Kaiho et al., 2022). Average combustion temperatures of normal wildfire is up to 700–1000 °C (including 300–1000 °C for grassland and leaf litter fires; Pyne et al., 1996; Goto, 1998) resulting in coronene index [coronene/(benzo[e]pyrene + beozo[ghi]perylene + coronene)] values <0.2 in normal sedimentary rocks (Kaiho et al., 2016a, Kaiho et al., 2021a, Kaiho et al., 2021b, Kaiho et al., 2022). While soil erosion events can cause enrichment of PAHs (Fox et al., 2022), they have low five- to seven-ringed PAHs produced by low-temperature (on average) combustion of wild fires (Kaiho et al., 2022). Higher values (> 0.3) observed from mass extinction horizons (Kaiho et al., 2016a, Kaiho et al., 2021a, Kaiho et al., 2021b), except for the end of the Triassic (Kaiho et al., 2022), are thought to reflect higher temperature combustion processes than average temperature of wildfires. Temperatures that are high enough to generate coronene are typically found only in large volatile volcanic events and asteroid−comet impacts (Kaiho et al., 2016a, Kaiho et al., 2021a, Kaiho et al., 2021b). Coincidental peaks of coronene and mercury, without an extraterrestrial iridium signature, can therefore be taken as more definitive evidence of high temperature volcanism (for Late Devonian and end-Permian mass extinctions; Kaiho et al., 2021a, Kaiho et al., 2021b). In contrast, low temperature volcanism is characterized by PAHs that have a dominance of benzo[e]pyrene and benzo[ghi]perylene instead of coronene (Kaiho et al., 2022). There has been no dominance of five- to seven-ringed benzo[e]pyrene, benzo[ghi]perylene, and coronene observed in non-event samples (Arinobu et al., 1999; Nabbefeld et al., 2010; Kaiho et al., 2016a, Kaiho et al., 2021a, Kaiho et al., 2021b, Kaiho et al., 2022; Lyons et al., 2020; Fox et al., 2022).
Soil erosion events have been detected in association with major mass extinctions during the F−F transition, D−C transition, end-Permian, end-Triassic, and the Cretaceous−Paleogene transition using dibenzofuran and n-alkanes (Sephton et al., 2005; Fenton et al., 2007; Mizukami et al., 2013, Mizukami et al., 2014; Kaiho et al., 2016a, Kaiho et al., 2016b, Kaiho et al., 2021a, Kaiho et al., 2021b, Kaiho et al., 2022; Biswas et al., 2020; Fox et al., 2022).
Here, we present analytical results of coronene, dibenzofuran, Hg, Mo, and carbonate carbon isotopes with Al and TOC from the GLB succession that records environmental and biotic crises in the GSSP Penglaitan section of the Laibin area, South China, and evaluate the causal relationship between the biotic crisis and enhanced volcanism at that time. We then use climate model results to explain why the terrestrial-nearshore crisis did not extend into the open marine realm during the G–L transition based on comparison with other major mass extinctions (Kaiho, 2022; Kaiho et al., 2021a).
_______________________________________________________________________________
RECURRING PATTERNS —
Mass extinction event 260 million years ago resulted from climate change, studies say
Ocean stagnation, ecosystem collapses, and volcano eruptions all played a role.
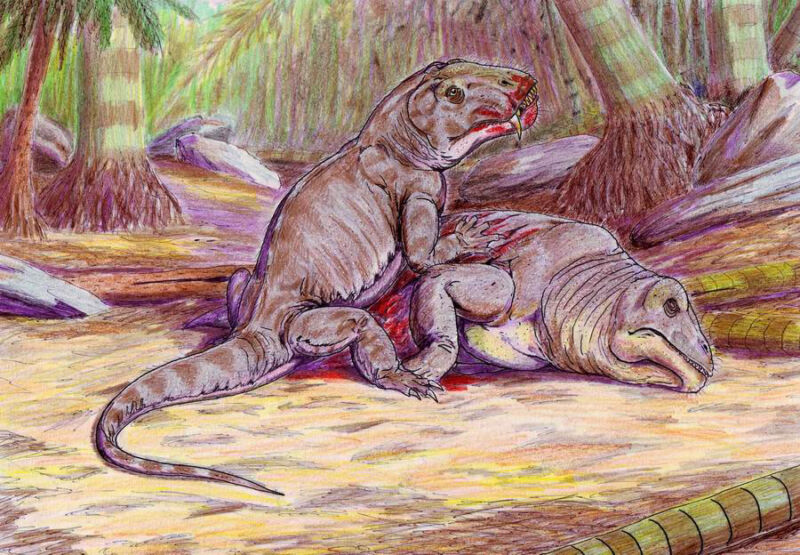
The Capitanian mass extinction was once lumped in with the “Great Dying” of the end-Permian mass extinction, but the lesser-known extinction occurred 8–10 million years earlier. It may not have been great, but it was quite lethal, seeing as many as 62 percent of species go extinct, according to one estimate. Two new papers by different teams shed new light on the event, revealing a pattern of cause and effect that’s seen in other mass extinctions: huge volcanic eruptions, global warming, the collapse of the terrestrial ecosystem, and the spread of oxygen-starved ocean dead zones. . .
Ocean dead zones. . .
Deforestation. . .
Destabilized climate. . .
A recurring pattern echoed today
- They all link to giant volcanism, the large igneous provinces.
- They all link with rapid global warming events as well, and
- There's the anoxia story as well, the ocean stagnation that you see happening at the same time,” said Wignall.
- “There are doubts about the timing of the biotic crisis, and the timing of the presumed LIP volcanism.
- All in all, a lot of uncertainties that clearly need more attention.”
- Instead of volcanic CO₂, we have CO₂ from fossil fuels, and we have methane leaks adding to global warming.
- Climate change is causing mass tree die-offs from droughts;
- anoxic ocean dead zones, while smaller than in the Capitanian, are spreading.
- Kaiho’s diagnosis of deforestation, deluges, and soil destruction are echoed today by the muddy floods this summer in the US, Japan, India, China, Turkey, Cuba, Italy, Spain, Chile, Brazil, Ivory Coast, and more.
Earth and Planetary Science Letters, 2023. DOI: doi.org/10.1016/j.epsl.2023.118128 (About DOIs).
Palaeogeography, Palaeoclimatology, Palaeoecology, 2023. DOI: doi.org/10.1016/j.palaeo.2023.111518
HOWARD LEEHoward Lee is a freelance science writer focusing on the evolution of planet Earth through deep time. He earned a B.Sc. in geology and M.Sc. in remote sensing, both from the University of London, UK.
_______________________________________________________________________________
No comments:
Post a Comment